Blog
Leveraging FRED for Advanced Thermal Imaging
Thermal imaging is an essential technology used in a wide array of applications, from defense and surveillance to environmental monitoring and industrial inspection. The ability to simulate thermal imaging systems accurately is crucial for optimizing their performance and reducing unwanted noise. In this blog post, we explore how FRED optical engineering software can be used to model and enhance thermal imaging systems, offering insights into noise management, reverse ray tracing, and thermal self-emission calculations.
Introduction to Thermal Imaging with FRED
Thermal imaging enables the detection and classification of objects based on their heat signature. This technology is pivotal for applications such as detecting concealed weapons, tracking personnel, and monitoring environmental conditions. FRED's advanced simulation capabilities allow for the precise modeling of thermal imaging systems, helping engineers design more effective and reliable systems.
One common example of thermal imaging in FRED is modeling a warm object, such as a teapot, imaged through a camera lens by an infrared detector. This basic setup is the foundation for more complex simulations.
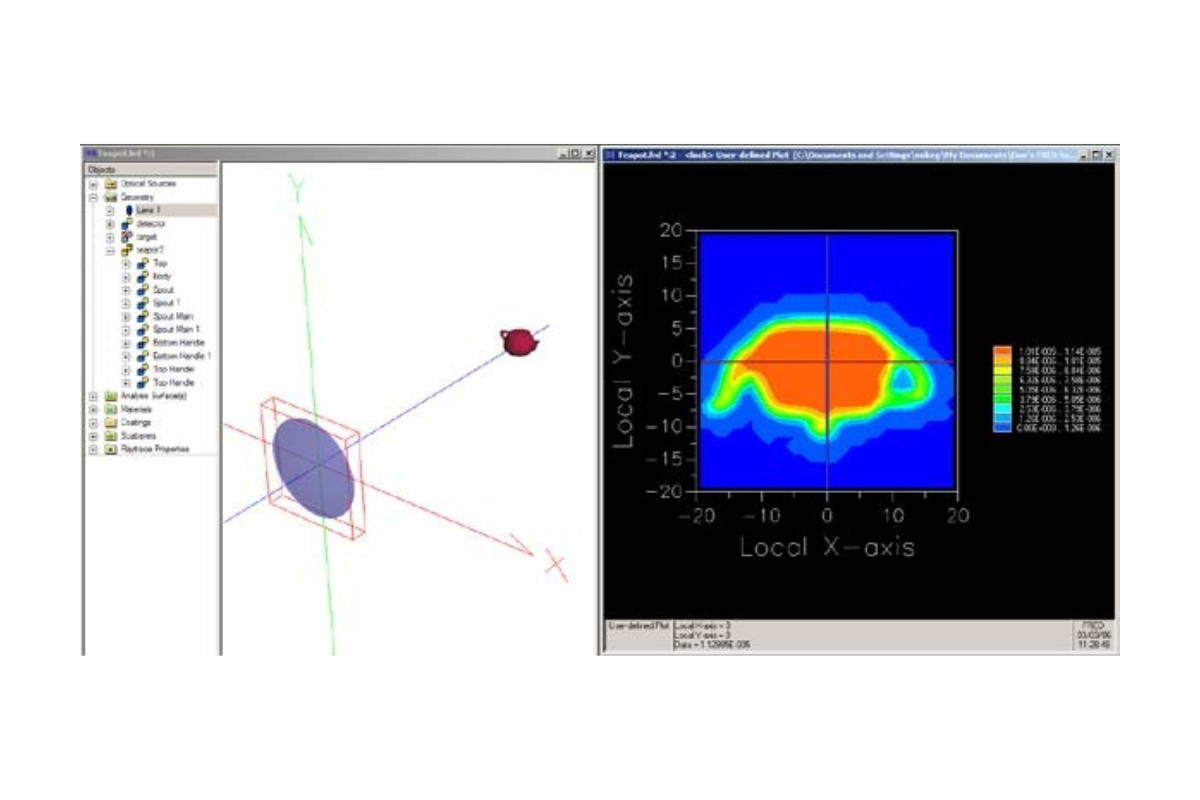
Figure 1: A warm teapot with different emissivities and temperature distributions is imaged through a singlet into a detector.
Managing Noise in Thermal Imaging
One of the key challenges in thermal imaging is dealing with noise, which can obscure the desired signal. Noise sources include background radiation, which needs to be subtracted to enhance contrast, and narcissus, a ghost image created by reflections within the optical system. FRED allows for detailed tracking of thermal emission paths, making it possible to identify and mitigate these noise sources.
Tracking Thermal Emission Paths
In FRED, an advanced raytrace can keep track of all ray paths within the system. This feature enables users to identify the contributions of different ray paths to the detector's signal, including those from ghosting, straight shots, and scattered rays.
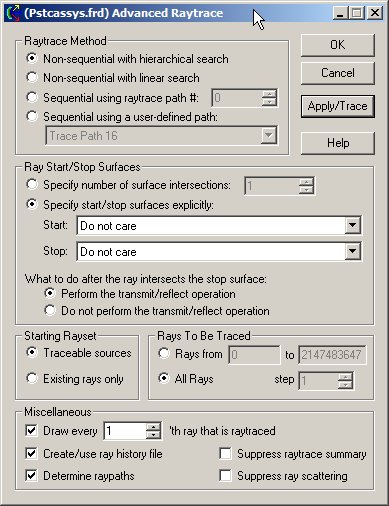
Figure 2: Advanced raytrace options in FRED, allowing for detailed tracking of ray paths.
By analyzing these paths, engineers can better understand how noise is introduced and how to optimize the system to reduce its impact.
Efficient Thermal Imaging Simulation with Reverse Ray Tracing
A highly efficient method for simulating thermal emission in FRED is reverse ray tracing. Instead of tracing rays from the source to the detector, reverse ray tracing starts at the detector and traces rays backward through the system. This approach significantly reduces the number of rays required to achieve accurate results, speeding up the simulation process.
Forward vs. Reverse Ray Tracing
A comparison between forward and reverse ray tracing in FRED shows that reverse ray tracing can achieve the same level of accuracy with far fewer rays, making it a preferred method for rapid assessments and iterative design processes.
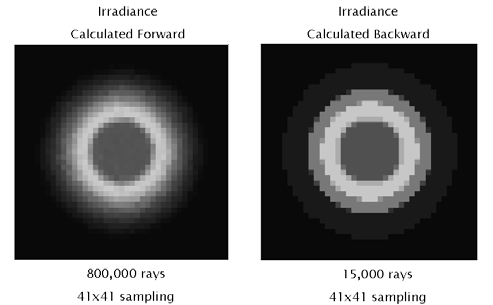
Figure 3: Comparison of irradiance calculations using forward and reverse ray tracing. Reverse ray tracing requires significantly fewer rays.
Quantifying Thermal Self-Emission
Thermal self-emission refers to the radiation emitted by objects within the optical system itself. If this radiation reaches the detector, it contributes to stray light, potentially degrading the system's performance. In FRED, thermal self-emission can be quantified using a combination of reverse ray tracing and radiometric principles.
Calculating Thermal Self-Emission
The thermal self-emission of a system can be calculated using the following equation:
TSE=∑(Ωobjectπ)ϵσT4Adetectorf(T)\text{TSE} = \sum \left(\frac{\Omega_{\text{object}}}{\pi}\right) \epsilon \sigma T^4 A_{\text{detector}} f(T)TSE=∑(πΩobject)ϵσT4Adetectorf(T)
Where:
- ϵ\epsilonϵ is the emissivity,
- σ\sigmaσ is the Stefan-Boltzmann constant,
- TTT is the temperature,
- AdetectorA_{\text{detector}}Adetector is the detector area, and
- Ωobject/π\Omega_{\text{object}}/\piΩobject/π is the projected solid angle of the object with respect to the detector.
By performing a reverse raytrace, the incident power on each object can be obtained, allowing for the calculation of the thermal self-emission for each component.
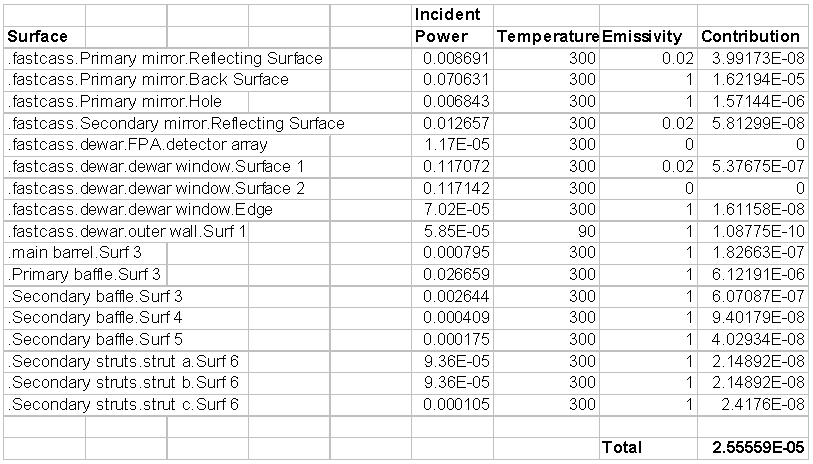
Figure 4: Example of calculating thermal self-emission for a Cassegrain telescope using FRED.
Conclusion
FRED provides powerful tools for simulating and optimizing thermal imaging systems. By leveraging advanced ray tracing techniques, such as reverse ray tracing, and applying radiometric concepts, engineers can accurately model thermal emission, manage noise, and improve the performance of thermal imaging devices. These capabilities make FRED an indispensable tool for anyone involved in the design and analysis of optical systems.
____________________________________________________________________________________________________________________________________________________________________________________
This blog post was created based on the information provided by Photon Engineering, a partner of CBS Europe.